Pharmacologic gut hormone modulation and eating behavior after esophagogastric cancer surgery: a narrative review
Introduction
Globally, metabolic disorders such as obesity and diabetes have reached epidemic status (1,2). Understanding the physiological, psychological and social factors that determine why we eat, what we eat and how much we eat is key in addressing obesity and its associated co-morbidities (3). Bariatric surgery has emerged as one of the most effective therapeutic interventions for metabolic diseases (4), and has enabled us to deepen our understanding of the mechanisms through which upper gastrointestinal (GI) surgery may affect energy homeostasis in overweight and obese populations, with gut hormones playing a pivotal role (5,6). Emerging evidence from normal weight cohorts undergoing upper GI cancer surgery, among whom weight maintenance is often a significant nutritional goal, has allowed us to develop a novel perspective on the changes in weight and eating behavior seen after surgery (7-9).
Physiologically, food intake is centrally controlled via complex networks that rely on the brain interpreting, integrating, and acting on signals of nutritional state and energy intake and expenditure (10). Achieving energy homeostasis involves a feedback loop comprising peripheral neuroendocrine signals, transmitted from the gut to the hypothalamus, to convey information about the nutritional state of the organism as well as nutrient availability. These afferent pathways are coupled with efferent signals, sent via vagal and spinal tracts, that modulate both ingestive behavior and energy expenditure (10-12). However, food intake is not only determined by homeostatic drivers. Neurobiological reward mechanisms, responding to palatable foods, comprise an additional element of control over nutritional intake, which also involves an integrated network of neuroendocrine signaling (13). Hedonic circuitry can override homeostatic signaling, leading to over-consumption of palatable foods (14). Thus, insights into the reward mechanisms that drive ingestive behavior are critical to understanding weight regulation in health and disease.
The GI tract plays a central role in the maintenance of energy homeostasis. Signals derived from the gut relay information regarding nutrient intake to the brain, initiating a response that manifests as changes in eating behavior. The interaction of ingested nutrients with the intestinal mucosa stimulates mucosal enteroendocrine cells to release gut hormones, which are considered key modulators of hunger and satiety signaling (11,15,16). A number of satiety gut hormones have now been identified, including glucagon-like peptide (GLP-1), peptide YY (PYY) and oxyntomodulin (OXM) which, when released from the enteroendocrine L cells, activate neurons in the subcortical areas of the brain to decrease appetite and increase satiation and satiety (15). In contrast, ghrelin represents the only known orexigenic gut hormone, responsible for stimulating hunger during periods of fasting (17).
Though attractively simple, eating behavior is not merely determined by oscillation in signals of hunger and satiety. This reductionist narrative does not acknowledge the role of food preference, food reward, and motivation to eat, commonly described as “appetitive” and “consummatory” behavior. Weight loss following upper GI surgery may therefore also be mediated by changes in eating behavior, presenting novel therapeutic targets. Increasing our mechanistic understanding of these alterations may enable the development of pharmacologic or nutrition-based strategies to optimize nutritional status. Bariatric surgery leads to exaggerated postprandial gut hormone secretion, including GLP-1, OXM, PYY, cholecystokinin (CCK), as well as attenuated postprandial pancreatic polypeptide (PP) secretion, of which GLP-1 and PYY have been studied most extensively and are associated with important changes in food reward, altered eating motivation, and body weight loss postoperatively (4,15,18-21). More recently, evidence has accrued reporting parallel findings following upper GI cancer surgery, with eating behavior amenable to pharmacologic manipulation (8,9,22-24).
This narrative review aims to explore the physiological mechanisms that determine eating behavior, discuss how this behavior is altered following esophagogastric cancer surgery, and identify pharmacologic therapies that can influence eating behavior to prevent malnutrition and mitigate weight loss. GLP-1 and PYY have been characterized most comprehensively in these contexts and the review therefore discusses postoperative gut hormone physiology using these hormones as markers of the postprandial satiety gut hormone response. The potential roles of other satiety gut hormones have been discussed in a previous review, together with other factors involved in postprandial gut-brain communication, such as bile acids and gut microbiota (25). The role of hormones signaling long-term systemic energy balance, such as leptin and insulin are not discussed herein.
We present the following article in accordance with the Narrative Review reporting checklist (available at http://dx.doi.org/10.21037/aoe-20-75).
Review methodology
A search of the recent literature using PubMed was undertaken using keywords including Eating behavior, Esophagectomy, Gastrectomy, Bariatric surgery, and Gut hormones. This yielded 592 results. Relevant studies identified through this search, as well as studies referenced in these articles, were reviewed, and 117 are referenced in this manuscript.
Central determinants of hedonic and homeostatic food intake
The neural circuitry of the gut-brain axis, can be further subdivided into homeostatic and hedonic pathways (26). In the homeostatic pathway, gut hormones relay information regarding dietary intake and peripheral energy levels to higher cortical centers of the brain (27). Located in the hypothalamus, the arcuate nucleus (ARC) is regarded as a key regulator of eating behavior and food intake (28,29). The satiety gut hormone GLP-1 partially exerts its effects via binding to GLP-1 receptors expressed in the ARC, which is crucial for mediating the effects of GLP-1 (30,31). Similarly, PYY3-36, one of two active forms of this hormone, is thought to exert its anorectic actions by stimulating inhibitory Y2 receptors located on a subset of ARC neurons expressing neuropeptide Y (NPY) and agouti-related peptide (AgRP) (32). On the other hand, receptors for the orexigenic hormone, ghrelin, are also located on NPY/AgRP (26,27,33). Circulating ghrelin activates these NPY/AgRP neurons, which, through the release of AgRP, inhibits melanocortin 3 and 4 receptors, and proopiomelanocortin cells, ultimately stimulating food intake (29,34). NPY/AgRP neurons also extend into adjacent hypothalamic nuclei, namely, the paraventricular nucleus, the dorsomedial nucleus and the lateral hypothalamic area, implicating these areas in homeostatic regulation (27,35,36).
The hedonic pathway, the other main system responsible for regulating food intake, operates in parallel with homeostatic signaling. This hedonic pathway is capable of overriding the nutrient requirements specified by the homeostatic pathway, and an interplay between both systems determines our unconscious approach to eating (26,28). Evidence suggests that the reward pathways of the limbic system, known to be stimulated by drugs of abuse, converge with pathways stimulated by highly palatable foods (37). Drugs of abuse typically increase dopamine signaling from the ventral tegmental area (VTA) that projects onto the nucleus accumbens (NAc). Regardless of their mechanism of action, these drugs all act upon the reward mechanism of the VTA-NAc pathway (26,37,38). This pathway has a clear evolutionary origin, mediating the positive emotional response to rewards essential to survival such as food and sex (39). Typically, dopamine release is tonic in nature. However, in response to salient environmental stimuli, such as food, a phasic (rapid firing) output of dopamine occurs, thus a reward cue is generated, drawing attention to the stimulus (40). The facilitation of behaviors directed towards returning to this reward primarily exists in the selective reinforcement of associations between a certain stimulus, and increased dopamine firing (41).
Crucially, appetitive drive, even towards food, is a learned behavior (42). In a murine study, mice that were incapable of producing dopamine, due to the selective inactivation of tyrosine hydroxylase in dopamine producing neurons, became profoundly hypophagic (43). In healthy humans, there is positive correlation between dopamine production in the dorsal striatum and the degree of pleasure experienced when eating food (44). In humans and rodents with obesity, a reduced availability of striatal D2 receptors has been observed (40). A study by Volkow et al. using PET imaging, demonstrated that striatal D2 receptor availability in subjects with obesity was significantly lower than normal weight participants (45). It was postulated that reduced dopamine receptor availability yielded an insufficient reward in response to a seemingly otherwise sufficient quantity of food, thus more food must be consumed in order to yield a desired response. Importantly, we know that modulation of palatability has a profound impact on perceived food reward, whereby animals will eat sweet and salty food past homeostatic requirement (13,28).
Evolutionarily, the ability to consume palatable foods beyond homeostatic need, allows for consumption when food is available, generating bodily stores to meet future requirements during times of environmental nutrient depletion. In modern Western society, with an abundant supply of food, our evolutionarily derived reward-signaling pathways can therefore lead to overeating and obesity (46). Studies have provided evidence for decreased dopamine production contributing to an inability to restrain behavior in drug-addiction, despite a conscious awareness of its negative effects (47). Similar findings are observed among individuals with morbid obesity (45). Disruption of D2 receptors in regions of the brain implicated in inhibitory control, namely the medial orbitofrontal cortex (OFC), cingulate gyrus and the dorsolateral prefrontal cortex, may lead to impulsive eating behavior, contributing to overeating as seen in obesity.
Eating behavior and the gut-brain axis
As outlined in Table 1, eating behaviour can be divided into appetitive, or food-seeking, and consummatory behavior. Although not mutually exclusive, distinguishing between these aspects is important when attempting to better understand the relationship between eating behavior and gut hormone signaling. Hunger, satiation and satiety, the latter two often erroneously used inter-changeably, are also defined for clarity. Unperturbed bi-directional communication between the GI tract and the brain is necessary for homeostatic maintenance of food intake (49). Gut hormones are paramount in regulating eating behavior, exerting their control via vagal afferents or acting directly on homeostatic brain centers through non-vagal afferents or the bloodstream (Figure 1) (11,27).
Table 1
Eating behavior | Definitions |
---|---|
Appetitive behavior | Is a behavior that increases the likelihood of consuming food perceived to be nutritious or decreases that of consuming potentially dangerous foods (48) |
Consummatory behavior | Is a behavior that promotes ingestive responses to, or prevents ingestion of foods taken into the mouth (48) |
Eating behavior | May be defined as: meal timing, quantity of food intake, and food preference, all the product of a complex interplay of physiologic signaling, psychological, social, and genetic factors (3) |
Hunger | Is a measurable, subjective impulse of an individual’s requirement to eat (3,18) |
Satiation | Is the process that terminates an eating episode, beginning when the gut signals fullness or when the person is satisfied with the amount consumed (18) |
Satiety | Is the period of time whereby an individual is satisfied they do not require food through the inhibition of hunger initiating mechanisms (18) |
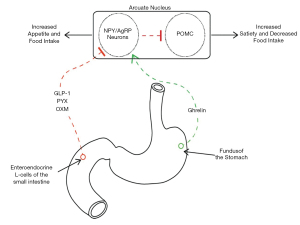
With regards to the regulation of food intake, gut hormones can be divided into two main classes: orexigenic (appetite-stimulating, such as ghrelin), and anorexigenic (satiety-stimulating, such as cholecystokinin (CCK), GLP-1, PYY, and OXM) (48). Ghrelin, a 28 amino-acid peptide, is secreted by Gr cells located predominantly in the gastric fundal mucosa, and stimulates hunger (50). Plasma concentrations of ghrelin are highest during periods of fasting, with a rapid postprandial decrease (51). PYY, GLP-1 and OXM are secreted postprandially by enteroendocrine L-cells, found throughout the small intestine, with higher concentrations distally (11). Collectively, this latter category of hormones stimulate satiation following a period of eating and contribute to the subsequent period of satiety.
A number of studies have now demonstrated exaggerated postprandial GLP-1 and PYY responses among patients following upper GI surgery, fueling an interest in investigating the role of these hormones in mediating food intake and weight loss in this context (9). GLP-1 is well known as a pleiotropic hormone, with multiple physiological actions, including inhibition of gastric emptying and gut motility, reduction of food intake, and an incretin effect-acting in concert with insulin to mediate postprandial glucose disposal (16,52-58). In addition to reducing overall food-intake, high levels of GLP-1 induce a conditioned taste aversion (CTA), a phenomenon whereby a learned negative association with a food results in a negative visceral response to that substance when it is encountered again (59). This may occur due to nausea in response to high circulating GLP-1 levels (52,60,61), as well as through effects on appetite centers in the brain. The mechanism by which GLP-1 induces CTA appears to be specific to particular nuclei in the brain, whereby administration of GLP-1 to both the lateral and 4th ventricles decrease food intake, but CTA only occurs when GLP-1 is administered to the lateral ventricle (61). It is worth noting however, that GLP-1 receptor knockout studies in mice do not produce an obese phenotype, which indicates an inbuilt redundancy in appetite regulation and highlights the complex nature of the physiological control of eating behavior (62,63). Notwithstanding the inherent nuance in certain contexts, antagonism of GLP-1 in satiated rats does increase food intake two-fold, suggesting that targeting GLP-1 may indeed be sufficient to influence energy balance (64).
Upper GI surgery, gut hormones and eating behavior
Bariatric surgery is an effective method of inducing and sustaining weight loss in patients with obesity (65). Roux-en-Y gastric bypass (RYGB) used since 1969 is recognized as an effective procedure to produce weight loss, achieving a mean postoperative body weight loss (BWL) of approximately 25-30% (4,18,19,66). Due to perceived surgical complexity of the RYGB, vertical sleeve gastrectomy (VSG) has increased in popularity, yielding broadly comparable outcomes (67,68).
Until recently, postoperative weight loss after RYGB and VSG was attributed to malabsorption or gastric restriction (18), however studies have demonstrated that these effects are not necessary nor sufficient to explain the mechanisms underlying the anorexigenic phenotype postoperatively. It is now well established that patients post bariatric surgery demonstrate significantly elevated postprandial levels of GLP-1, PYY, OXM, CCK, while bile acids are also exaggerated (Figure 2) (4,9,15,18,19,66,69-72). Patients after RYGB and VSG typically exhibit greater weight loss when compared to those undergoing procedures such as gastric band surgery, with the latter not associated with significant alterations in satiety gut hormones, albeit that signaling after the band may be associated with vagal changes (4).
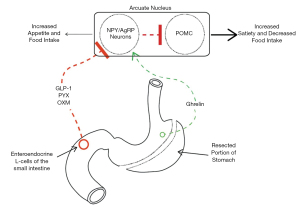
Eating behavior changes after bariatric surgery, with patients reporting reduced calorie intake, though, interestingly, food preference has also been reported to shift from calorie-dense and sweet foods to healthier alternatives (73,74). Although this finding may be confounded by dietary advice received by patients post bariatric surgery, namely to improve diet quality and reduce calorie intake, these findings have been shown in rodent models (75). Studies are on-going to elucidate whether this impact on macronutrient preference is evident post oesophagogastric cancer surgery, where patients are not asked to follow low-calorie diets postoperatively. The differential impact of these varying operative approaches may be explained, at least partially, by variable effects on both hedonic and homeostatic regulation (19).
Drawing from this bariatric research, the role of gut hormones as mediators of weight loss following esophagectomy or gastrectomy for cancer has become a topic of growing academic interest (8,9,22,23,76). Like bariatric surgery, these cancer operations involve major reconstruction of the upper GI tract (Figure 3). Approximately 33% of recurrence-free esophagectomy patients demonstrate postoperative BWL of over 15%, associated with impaired long-term nutritional status and quality of life (76-82). This unintentional weight loss is also associated with exaggerated postprandial gut hormone secretion in the long-term after surgery (9,83).
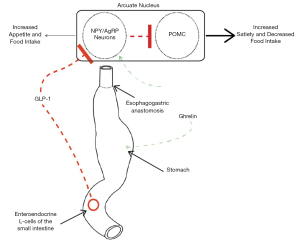
To summarize, postprandial release of satiety hormones stimulate satiation and satiety, and an exaggerated pattern is clearly evident after upper GI surgery (4,19,66,67,72,84,85). The anorectic effects of upper GI surgery may therefore be due, in part, to aberrant gut-brain signaling. These data suggest that postoperative weight loss is more complex and nuanced than the previously held dogma asserting that gastric restriction and nutrient malabsorption are solely responsible. They may also have significant implications with regards to the mitigation of unintentional weight loss after esophagogastric surgery.
Bariatric surgery and eating behavior
Patients after bariatric surgery show a reduction in meal sizes, while some also show a reduction in dietary energy density, that is, the amount of kilocalories per kilogram of food (18,86,87). Fat has the largest influence on calorie intake, given that its energy density is 9 versus 4 kcal/g for protein or carbohydrate (88). There is evidence that taste preference is significantly altered following bariatric surgery and that these changes correlate with a reduction in postoperative body mass index (BMI) (89). A 2-year follow up study by Coluzzi et al., reported that following laparoscopic sleeve gastrectomy, 75% of patients displayed a reduced interest and taste for sweets and high-fat foods (73). A study by le Roux et al., compared dietary fat intake between two groups of patients, one having had RYGB and another who underwent vertical-banded gastroplasty (a procedure associated with no significant change in postprandial gut hormone signaling) (75). With no significant difference in percentage of fat intake between the two groups prior to their respective surgeries, the RYGB group consumed less fat in their diet compared with the vertical-banded gastroplasty group, 1- and 6-year post-surgery. Similarly, data suggest that the reward value of sweet and fatty foods is diminished in adolescents who have undergone VSG as an intervention for weight loss (90,91). This suggests that bariatric procedures such as VSG and RYGB not only reduce food intake through gut hormone alterations, but may also alter hedonic eating, shifting macronutrient selection towards low energy foods.
The relationship between exaggerated GLP-1 secretion and eating behavior has also been investigated. When gut hormone secretion is suppressed using a somatostatin analog in patients post bariatric surgery, motivation to eat and hedonic signaling increase (20). These results may implicate GLP-1 as a mediator of macronutrient selection. Using fMRI, eating behavior was monitored in two cohorts, one with a history of RYGB and the other, post gastric band surgery. BOLD changes in reward centers of the brain were compared between groups in response to food images. In comparison to the band group, RYGB patients displayed a decreased activation in several reward centers in response to high-calorie foods (19).
Fundamentally, the ability of hedonic signaling to override homeostatically-determined nutritional requirements may be a causative factor in overeating, contributing to obesity. Both appetitive and consummatory behavior are influenced by bariatric surgery, particularly RYGB and VSG, and may be important in mediating postoperative weight loss (6).
Esophagectomy and eating behavior
While weight loss is a common presenting complaint in esophageal cancer, this is often exacerbated following resection, and commonly persists even among disease-free patients (9,76,92,93), with patients are at an increased risk of malnutrition (76). Prolonged malnutrition in this cohort can severely impact recovery of health-related quality of life in survivorship (94). Insights into the mechanisms underlying weight loss among patients post bariatric surgery have led to the investigation of whether similar underlying mechanisms may be responsible for weight loss in patients undergoing esophagectomy (22).
A study by Koizumi et al., explored the role of the hunger hormone ghrelin in postoperative weight loss after esophagectomy. Although there was an initial marked reduction in circulating ghrelin levels after esophagectomy (95), levels returned to preoperative concentrations approximately 3 months post-surgery, facilitating the return of hunger. These findings have been since replicated, with ghrelin concentrations recovering by 12 months postoperatively in a prospective study of patients undergoing esophagectomy with gastric conduit reconstruction (83). Despite often describing a restored appetite, or specifically hunger, patients often report that they are unable to finish a meal due to a premature feeling of ‘fullness’ or due to significant postprandial symptoms (93,95). This suggests that anorexigenic, or satiety, hormones may play a more significant role in long-term postoperative nutritional impairment in the long term.
In a prospective study by Elliott et al., weight loss, satiety, and postprandial gut hormone response were analyzed pre-and postoperatively in patients who underwent uncomplicated esophagectomy with gastric conduit reconstruction and pyloroplasty (9). In this study, plasma GLP-1 was measured before and after a standardized 400-kcal mixed meal challenge at serial timepoints. Patients displayed progressive weight loss at 6 weeks and 3 months postoperatively. This correlated with a significant, exaggerated postprandial increase in plasma levels of GLP-1 10 days, 6 weeks, and 3 months postoperatively. The authors noted a relationship between the exaggerated GLP-1 response and self-reported post-ingestive symptoms, which were increased following surgery, with 62% reporting clinically significant symptoms of “dumping syndrome”. “Dumping syndrome”, a phenomenon that is prevalent after esophageal surgery (96), can also itself influence eating behavior by inducing a conditioned food avoidance (59). In this setting, patients often describe limiting food intake to prevent or reduce such unwanted post-ingestive symptoms. The same cohort was subsequently followed until the 12-month postoperative timepoint, with the exaggerated GLP-1 response and weight loss persisting, and postprandial hypoglycemia emerging as another nutritional consequence of esophageal surgery that could potentially influence eating behavior (83). These findings may implicate gut hormones as key mediators of altered eating behavior after esophagectomy.
Gut hormone-targeted interventions to modulate eating behavior after upper GI cancer surgery
The discovery that parallel changes in gut hormone secretion occur in diverse upper GI surgical contexts has led to the hypothesis that pharmacologic suppression of these mediators may prevent or mitigate weight loss after upper GI cancer surgery. Although manipulation of circulating ghrelin has been explored, therapeutic benefit to date has been limited. A randomized placebo controlled phase II clinical study by Yamamoto et al., showed that exogenous administration of ghrelin prior to a meal stimulated food intake and attenuated initial postoperative weight loss in the early postoperative setting (97). However, whether this translates to improved long-term nutritional outcomes is yet to be established, while the persistence of reduced body weight and altered eating behavior despite the normalization of endogenous ghrelin production during the first postoperative year suggests that satiety gut hormones may represent a more viable therapeutic target in this context.
In this regard, somatostatin is a regulatory peptide produced by enteroendocrine D cells in the GI tract that acts to suppress gut hormones in a negative feedback loop, maintaining homeostasis in appetitive signaling (98). Therefore, it has been studied as a potential therapeutic to modulate eating behavior among patients following esophagectomy. Somatostatin delays gastric emptying and intestinal transit, and directly suppresses the release of satiety gut hormones and insulin, while reducing postprandial splanchnic vasodilation by inhibiting the release of vasoactive factors (99-108). Theoretically, therefore, its actions should specifically target and mitigate the spectrum of symptoms that manifest after esophagectomy, minimizing the emergence of conditioned avoidance of certain foods. A variety of somatostatin analogs have, in fact, been studied in the context of dumping syndrome post gastrectomy, with varying degrees of success (109-113). In a placebo-controlled trial, among patients who had undergone gastric resection, treatment with somatostatin prevented the onset of early dumping syndrome symptoms following a glucose provocation test when compared with placebo (110). In another small study of patients after gastric surgery who were challenged with a 50% glucose drink, intravenous somatostatin prevented an increase in pulse rate and the onset of diarrhea (109). Various placebo-controlled trials have also demonstrated that long acting octreotide is an effective treatment in the prevention of early dumping syndrome symptoms among post-gastrectomy patients (111-112). Additionally, octreotide has been effective in the prevention of postprandial hypoglycemia following a high carbohydrate meal challenge when compared to placebo after upper GI surgery (112). However, until recently, studies have not focused on changes in eating behavior or nutritional status.
Among post-esophagectomy cohorts given a single dose of the somatostatin analog octreotide, recent work highlights a number of key observations. In a randomized controlled trial, octreotide suppressed gut hormones among patients post esophagectomy, and led to a four-fold increase in ad libitum food intake relative to placebo and to unoperated control patients, in whom the drug had no effect (8). This increased caloric intake was seen without any change in postprandial fullness or nausea. Moreover, in another randomized study of disease-free patients post esophagectomy, octreotide directly influenced appetitive behavior with acute gut hormone suppression driving an increase in motivation to eat (24). Thus, in this context, attenuating gut hormone release appears to modulate eating behavior and may serve as a novel therapeutic approach for longer term treatment.
Limitations and quality of evidence
It is reasonable to conclude that given the early success of gut hormone-suppressing agents there may be potential for wider application as part of a multimodal approach towards nutritional optimization of patients with an exaggerated gut hormone response. However, it is important to acknowledge the limitations of the available evidence. The aforementioned studies only demonstrate the effect of gut hormone suppression in single meal or short-term settings (8,20,24). Moreover, sample sizes in studies to date that explore pharmacologic gut hormone modulation in these postoperative contexts are small (8,9,24,83). Notwithstanding, evidence exploring the role of short-term gut hormone suppression post oesophagogastric surgery are randomized and placebo-controlled and, as such, generally of high quality (8,20,24). Ultimately, despite therapeutic promise, there remains overall a paucity of empirical evidence regarding the impact of longer-term gut hormone suppression on eating behavior, nutritional outcomes, and health-related quality of life.
Conclusion
Upper GI surgery has significant effects on eating behavior. This may be linked to altered gut-brain axis signaling postoperatively, acting via both homeostatic and hedonic pathways. In the setting of esophageal cancer surgery specifically, the significant burden of malnutrition and associated quality of life impact may be ameliorated by suppressing the exaggerated gut hormone signaling to improve eating behavior. Eating behavior encapsulates appetitive behavior and macronutrient selection, as well as meal size and frequency. Given the role of gut hormones in not only satiation and satiety, but also in the pathophysiology of post-ingestive symptoms, which may drive food selection and conditioned taste aversion, targeting gut hormones may restore appropriate eating behavior through several pathways. Critically, therapeutic approaches in future should recognize the distinct aspects of eating behavior, which will enable appropriate targeting of therapy to patients based on individual deficits. Taking a more nuanced view of energy intake determinants, beyond the homeostatic drivers, that incorporates hedonic factors will aid the investigation and development of promising therapies. Moreover, understanding how emerging therapies with narrower spectra of gut hormone suppression affect eating behavior in this context may enable targeting of treatment based on the specific alterations seen in individuals postoperatively.
In conclusion, gut hormone directed therapeutics may represent a novel strategy for the optimization of nutritional status following esophagogastric surgery. Further studies should assess the long-term impact of gut hormone modulation on nutrition and health-related quality of life among patients with nutritional compromise following esophagogastric surgery.
Acknowledgments
Funding: None.
Footnote
Reporting Checklist: The authors have completed the Narrative Review reporting checklist. Available at http://dx.doi.org/10.21037/aoe-20-75
Conflicts of Interest: All authors have completed the ICMJE uniform disclosure form (available at http://dx.doi.org/10.21037/aoe-20-75). JVR serves as an unpaid editorial board member of Annals of Esophagus from September 2019 to August 2023. The other authors have no conflicts of interest to declare.
Ethical Statement: The authors are accountable for all aspects of the work in ensuring that questions related to the accuracy or integrity of any part of the work are appropriately investigated and resolved.
Open Access Statement: This is an Open Access article distributed in accordance with the Creative Commons Attribution-NonCommercial-NoDerivs 4.0 International License (CC BY-NC-ND 4.0), which permits the non-commercial replication and distribution of the article with the strict proviso that no changes or edits are made and the original work is properly cited (including links to both the formal publication through the relevant DOI and the license). See: https://creativecommons.org/licenses/by-nc-nd/4.0/.
References
- James PT. Obesity: the worldwide epidemic. Clin Dermatol 2004;22:276-80. [Crossref] [PubMed]
- Troke RC, Tan TM, Bloom SR. The future role of gut hormones in the treatment of obesity. Ther Adv Chronic Dis 2014;5:4-14. [Crossref] [PubMed]
- Grimm ER, Steinle NI. Genetics of eating behavior: established and emerging concepts. Nutr Rev 2011;69:52-60. [Crossref] [PubMed]
- Spector AC, Kapoor N, Price RK, et al. Proceedings from the 2018 Association for Chemoreception Annual Meeting Symposium: Bariatric Surgery and Its Effects on Taste and Food Selection. Chem Senses 2019;44:155-63.
- le Roux CW, Bueter M. The physiology of altered eating behaviour after Roux-en-Y gastric bypass. Exp Physiol 2014;99:1128-32. [Crossref] [PubMed]
- Miras AD, le Roux CW. Mechanisms underlying weight loss after bariatric surgery. Nat Rev Gastroenterol Hepatol 2013;10:575-84. [Crossref] [PubMed]
- Roberts GP, Kay RG, Howard J, et al. Gastrectomy with Roux-en-Y reconstruction as a lean model of bariatric surgery. Surgery for obesity and related diseases 2018;14:562-8. [Crossref] [PubMed]
- Elliott JA, Jackson S, King S, et al. Gut Hormone Suppression Increases Food Intake After Esophagectomy With Gastric Conduit Reconstruction. Ann Surg 2015;262:824-9. [Crossref] [PubMed]
- Elliott JA, Docherty NG, Eckhardt HG, et al. Weight Loss, Satiety, and the Postprandial Gut Hormone Response After Esophagectomy: A Prospective Study. Ann Surg 2017;266:82-90. [Crossref] [PubMed]
- Magni P, Dozio E, Ruscica M, et al. Feeding behavior in mammals including humans. Ann N Y Acad Sci 2009;1163:221-32. [Crossref] [PubMed]
- Sam AH, Troke RC, Tan TM, et al. The role of the gut/brain axis in modulating food intake. Neuropharmacology 2012;63:46-56. [Crossref] [PubMed]
- Williams G, Bing C, Cai XJ, et al. The hypothalamus and the control of energy homeostasis: different circuits, different purposes. Physiol Behav 2001;74:683-701. [Crossref] [PubMed]
- Volkow ND, Wang GJ, Baler RD. Reward, dopamine and the control of food intake: implications for obesity. Trends Cogn Sci 2011;15:37-46. [Crossref] [PubMed]
- Egecioglu E, Skibicka KP, Hansson C, et al. Hedonic and incentive signals for body weight control. Rev Endocr Metab Disord 2011;12:141-51. [Crossref] [PubMed]
- Perry B, Wang Y. Appetite regulation and weight control: the role of gut hormones. Nutr Diabetes 2012;2:e26. [Crossref] [PubMed]
- Lim GE, Brubaker PL. Glucagon-like peptide 1 secretion by the L-cell - The view from within. Diabetes 2006;55:S70-7. [Crossref]
- Miyazaki T, Tanaka N, Hirai H, et al. Ghrelin level and body weight loss after esophagectomy for esophageal cancer. J Surg Res 2012;176:74-8. [Crossref] [PubMed]
- Al-Najim W, Docherty NG, le Roux CW. Food Intake and Eating Behavior After Bariatric Surgery. Physiol Rev 2018;98:1113-41. [Crossref] [PubMed]
- Scholtz S, Miras AD, Chhina N, et al. Obese patients after gastric bypass surgery have lower brain-hedonic responses to food than after gastric banding. Gut 2014;63:891-902. [Crossref] [PubMed]
- Goldstone AP, Miras AD, Scholtz S, et al. Link Between Increased Satiety Gut Hormones and Reduced Food Reward After Gastric Bypass Surgery for Obesity. J Clin Endocrinol Metab 2016;101:599-609. [Crossref] [PubMed]
- Miras AD, Jackson RN, Jackson SN, et al. Gastric bypass surgery for obesity decreases the reward value of a sweet-fat stimulus as assessed in a progressive ratio task. Am J Clin Nutr 2012;96:467-73. [Crossref] [PubMed]
- Murphy C. The potential mechanisms of unintentional weight loss after oesophageal cancer surgery. CAB Reviews: Perspectives in Agriculture, Veterinary Science, Nutrition and Natural Resources 2018;13:043.
- Taguchi M, Dezaki K, Koizumi M, et al. Total gastrectomy-induced reductions in food intake and weight are counteracted by rikkunshito by attenuating glucagon-like peptide-1 elevation in rats. Surgery 2016;159:1342-50. [Crossref] [PubMed]
- Elliott JA, Docherty NG, Haag J, et al. Attenuation of satiety gut hormones increases appetitive behavior after curative esophagectomy for esophageal cancer. Am J Clin Nutr 2019;109:335-44. [Crossref] [PubMed]
- Elliott JA, Reynolds JV, le Roux CW, et al. Physiology, pathophysiology and therapeutic implications of enteroendocrine control of food intake. Expert Rev Endocrinol Metab 2016;11:475-99. [Crossref] [PubMed]
- Lutter M, Nestler EJ. Homeostatic and hedonic signals interact in the regulation of food intake. J Nutr 2009;139:629-32. [Crossref] [PubMed]
- Buhmann H, le Roux CW, Bueter M. The gut-brain axis in obesity. Best Pract Res Clin Gastroenterol 2014;28:559-71. [Crossref] [PubMed]
- Saper CB, Chou TC, Elmquist JK. The need to feed: homeostatic and hedonic control of eating. Neuron 2002;36:199-211. [Crossref] [PubMed]
- Waterson MJ, Horvath TL. Neuronal Regulation of Energy Homeostasis: Beyond the Hypothalamus and Feeding. Cell Metab 2015;22:962-70. [Crossref] [PubMed]
- Göke R, Larsen PJ, Mikkelsen JD, et al. Distribution of Glp-1 Binding-Sites in the Rat-Brain - Evidence That Exendin-4 Is a Ligand of Brain Glp-1 Binding-Sites. Eur J Neurosci 1995;7:2294-300. [Crossref] [PubMed]
- Tang-Christensen M, Vrang N, Larsen PJ. Glucagon-like peptide 1(7-36) amide's central inhibition of feeding and peripheral inhibition of drinking are abolished by neonatal monosodium glutamate treatment. Diabetes 1998;47:530-7. [Crossref] [PubMed]
- Batterham RL, Cowley MA, Small CJ, et al. Gut hormone PYY3-36 physiologically inhibits food intake. Nature 2002;418:650-4. [Crossref] [PubMed]
- Schwartz MW, Woods SC, Porte D Jr, et al. Central nervous system control of food intake. Nature 2000;404:661-71. [Crossref] [PubMed]
- Mountjoy KG. Pro-Opiomelanocortin (POMC) Neurones, POMC-Derived Peptides, Melanocortin Receptors and Obesity: How Understanding of this System has Changed Over the Last Decade. J Neuroendocrinol 2015;27:406-18. [Crossref] [PubMed]
- Suzuki K, Jayasena CN, Bloom SR. Obesity and appetite control. Exp Diabetes Res 2012;2012:824305. [Crossref] [PubMed]
- Heisler LK, Lam DD. An appetite for life: brain regulation of hunger and satiety. Curr Opin Pharmacol 2017;37:100-6. [Crossref] [PubMed]
- Nestler EJ. Is there a common molecular pathway for addiction? Nat Neurosci 2005;8:1445-9. [Crossref] [PubMed]
- Wise RA. Dopamine, learning and motivation. Nat Rev Neurosci 2004;5:483-94. [Crossref] [PubMed]
- Kelley AE, Berridge KC. The neuroscience of natural rewards: relevance to addictive drugs. J Neurosci 2002;22:3306-11. [Crossref] [PubMed]
- Palmiter RD. Is dopamine a physiologically relevant mediator of feeding behavior? Trends Neurosci 2007;30:375-81. [Crossref] [PubMed]
- Arias-Carrión O, Stamelou M, Murillo-Rodriguez E, et al. Dopaminergic reward system: a short integrative review. Int Arch Med 2010;3:24. [Crossref] [PubMed]
- Konno K, Terashima H, Miyano N, et al. Nihon Shokakibyo Gakkai Zasshi 1994;91:1067-72. [A case of descending colon stenosis associated with acute pancreatitis]. [PubMed]
- Szczypka MS, Kwok K, Brot MD, et al. Dopamine production in the caudate putamen restores feeding in dopamine-deficient mice. Neuron 2001;30:819-28. [Crossref] [PubMed]
- Small DM, Jones-Gotman M, Dagher A. Feeding-induced dopamine release in dorsal striatum correlates with meal pleasantness ratings in healthy human volunteers. Neuroimage 2003;19:1709-15. [Crossref] [PubMed]
- Volkow ND, Wang GJ, Telang F, et al. Low dopamine striatal D2 receptors are associated with prefrontal metabolism in obese subjects: possible contributing factors. Neuroimage 2008;42:1537-43. [Crossref] [PubMed]
- Johnson PM, Kenny PJ. Dopamine D2 receptors in addiction-like reward dysfunction and compulsive eating in obese rats. Nat Neurosci 2010;13:635-41. [Crossref] [PubMed]
- Volkow ND, Wang GJ, Telang F, et al. Profound decreases in dopamine release in striatum in detoxified alcoholics: possible orbitofrontal involvement. J Neurosci 2007;27:12700-6. [Crossref] [PubMed]
- Zanchi D, Depoorter A, Egloff L, et al. The impact of gut hormones on the neural circuit of appetite and satiety: A systematic review. Neurosci Biobehav Rev 2017;80:457-75. [Crossref] [PubMed]
- Cryan JF, O'Mahony SM. The microbiome-gut-brain axis: from bowel to behavior. Neurogastroenterol Motil 2011;23:187-92. [Crossref] [PubMed]
- Murakami N, Hayashida T, Kuroiwa T, et al. Role for central ghrelin in food intake and secretion profile of stomach ghrelin in rats. J Endocrinol 2002;174:283-8. [Crossref] [PubMed]
- Cummings DE, Purnell JQ, Frayo RS, et al. A preprandial rise in plasma ghrelin levels suggests a role in meal initiation in humans. Diabetes 2001;50:1714-9. [Crossref] [PubMed]
- Shah M, Vella A. Effects of GLP-1 on appetite and weight. Rev Endocr Metab Disord 2014;15:181-7. [Crossref] [PubMed]
- Flint A, Raben A, Astrup A, et al. Glucagon-like peptide 1 promotes satiety and suppresses energy intake in humans. J Clin Invest 1998;101:515-20. [Crossref] [PubMed]
- Verdich C, Flint A, Gutzwiller JP, et al. A meta-analysis of the effect of glucagon-like peptide-1 (7-36) amide on ad libitum energy intake in humans. J Clin Endocrinol Metab 2001;86:4382-9. [PubMed]
- De Silva A, Salem V, Long CJ, et al. The gut hormones PYY 3-36 and GLP-1 7-36 amide reduce food intake and modulate brain activity in appetite centers in humans. Cell Metab 2011;14:700-6. [Crossref] [PubMed]
- Näslund E, Barkeling B, King N, et al. Energy intake and appetite are suppressed by glucagon-like peptide-1 (GLP-1) in obese men. Int J Obes Relat Metab Disord 1999;23:304-11. [Crossref] [PubMed]
- Baggio LL, Drucker DJ. Biology of incretins: GLP-1 and GIP. Gastroenterology 2007;132:2131-57. [Crossref] [PubMed]
- Holst JJ. The physiology of glucagon-like peptide 1. Physiol Rev 2007;87:1409-39. [Crossref] [PubMed]
- Schier LA, Spector AC. The functional and neurobiological properties of bad taste. Physiol Rev 2019;99:605-63. [Crossref] [PubMed]
- Dailey MJ, Moran TH. Glucagon-like peptide 1 and appetite. Trends Endocrinol Metab 2013;24:85-91. [Crossref] [PubMed]
- Thiele TE, van Dijk G, Campfield AL, et al. Central Infusion of GLP-1, but not Leptin, Produces Conditioned Taste Aversions in Rats. Am J Physiol 1997;272:R726-30. [PubMed]
- Schjoldager BT, Mortensen PE, Christiansen J, et al. GLP-1 (glucagon-like peptide 1) and truncated GLP-1, fragments of human proglucagon, inhibit gastric acid secretion in humans. Dig Dis Sci 1989;34:703-8. [Crossref] [PubMed]
- Day JW, Ottaway N, Patterson JT, et al. A new glucagon and GLP-1 co-agonist eliminates obesity in rodents. Nat Chem Biol 2009;5:749-57. [Crossref] [PubMed]
- Turton MD, O'Shea D, Gunn I, et al. A role for glucagon-like peptide-1 in the central regulation of feeding. Nature 1996;379:69-72. [Crossref] [PubMed]
- Mann J, Truswell AS. Essentials of human nutrition. vol 4. United States: Oxford University Press Inc.; 2012.
- Chambers AP, Smith EP, Begg DP, et al. Regulation of gastric emptying rate and its role in nutrient-induced GLP-1 secretion in rats after vertical sleeve gastrectomy. Am J Physiol Endocrinol Metab 2014;306:E424-32. [Crossref] [PubMed]
- Chambers AP, Stefater MA, Wilson-Perez HE, et al. Similar effects of roux-en-Y gastric bypass and vertical sleeve gastrectomy on glucose regulation in rats. Physiol Behav 2011;105:120-3. [Crossref] [PubMed]
- Lakdawala MA, Bhasker A, Mulchandani D, et al. Comparison between the results of laparoscopic sleeve gastrectomy and laparoscopic Roux-en-Y gastric bypass in the Indian population: a retrospective 1 year study. Obes Surg 2010;20:1-6. [Crossref] [PubMed]
- Wallenius V, Dirinck E, Fändriks L, et al. Glycemic Control after Sleeve Gastrectomy and Roux-En-Y Gastric Bypass in Obese Subjects with Type 2 Diabetes Mellitus. Obes Surg 2018;28:1461-72. [Crossref] [PubMed]
- Dimitriadis GK, Randeva MS, Miras AD. Potential Hormone Mechanisms of Bariatric Surgery. Curr Obes Rep 2017;6:253-65. [Crossref] [PubMed]
- Beckman LM, Beckman TR, Sibley SD, et al. Changes in gastrointestinal hormones and leptin after Roux-en-Y gastric bypass surgery. JPEN J Parenter Enteral Nutr 2011;35:169-80. [Crossref] [PubMed]
- Holdstock C, Zethelius B, Sundbom M, et al. Postprandial changes in gut regulatory peptides in gastric bypass patients. Int J Obes (Lond) 2008;32:1640-6. [Crossref] [PubMed]
- Coluzzi I, Raparelli L, Guarnacci L, et al. Food Intake and Changes in Eating Behavior After Laparoscopic Sleeve Gastrectomy. Obes Surg 2016;26:2059-67. [Crossref] [PubMed]
- Gero D, Steinert RE, le Roux CW, et al. Do Food Preferences Change After Bariatric Surgery? Curr Atheroscler Rep 2017;19:38. [Crossref] [PubMed]
- le Roux CW, Bueter M, Theis N, et al. Gastric bypass reduces fat intake and preference. Am J Physiol Regul Integr Comp Physiol 2011;301:R1057-66. [Crossref] [PubMed]
- Martin L, Lagergren P. Long-term weight change after oesophageal cancer surgery. Br J Surg 2009;96:1308-14. [Crossref] [PubMed]
- Markar SR, Zaninotto G, Castoro C, et al. Lasting Symptoms After Esophageal Resection (LASER): European Multicenter Cross-sectional Study. Ann Surg 2020. [Epub ahead of print].
- Schandl A, Kauppila JH, Anandavadivelan P, et al. Predicting the Risk of Weight Loss After Esophageal Cancer Surgery. Ann Surg Oncol 2019;26:2385-91. [Crossref] [PubMed]
- Hellstadius Y, Malmström M, Lagergren P, et al. Reflecting a crisis reaction: Narratives from patients with oesophageal cancer about the first 6 months after diagnosis and surgery. Nursing Open 2019;6:1471-80. [Crossref] [PubMed]
- Anandavadivelan P, Wikman A, Johar A, et al. Impact of weight loss and eating difficulties on health‐related quality of life up to 10 years after oesophagectomy for cancer. Br J Surg 2018;105:410-8. [Crossref] [PubMed]
- Djärv T, Blazeby JM, Lagergren P. Predictors of postoperative quality of life after esophagectomy for cancer. J Clin Oncol 2009;27:1963-8. [Crossref] [PubMed]
- Martin L, Lagergren J, Lindblad M, et al. Malnutrition after oesophageal cancer surgery in Sweden. Br J Surg 2007;94:1496-500. [Crossref] [PubMed]
- Elliott JA, Docherty N, Murphy C, et al. Changes in gut hormones, glycaemic response and symptoms after oesophagectomy. Br J Surg 2019;106:735-46. [Crossref] [PubMed]
- Dar MS, Chapman WH 3rd, Pender JR, et al. GLP-1 response to a mixed meal: what happens 10 years after Roux-en-Y gastric bypass (RYGB)? Obes Surg 2012;22:1077-83. [Crossref] [PubMed]
- Vigneshwaran B, Wahal A, Aggarwal S, et al. Impact of Sleeve Gastrectomy on Type 2 Diabetes Mellitus, Gastric Emptying Time, Glucagon-Like Peptide 1 (GLP-1), Ghrelin and Leptin in Non-morbidly Obese Subjects with BMI 30-35.0 kg/m(2): a Prospective Study. Obes Surg 2016;26:2817-23. [Crossref] [PubMed]
- Ello-Martin JA, Roe LS, Ledikwe JH, et al. Dietary energy density in the treatment of obesity: a year-long trial comparing 2 weight-loss diets. Am J Clin Nutr 2007;85:1465-77. [Crossref] [PubMed]
- Søndergaard Nielsen M, Rasmussen S, Just Christensen B, et al. Bariatric Surgery Does Not Affect Food Preferences, but Individual Changes in Food Preferences May Predict Weight Loss. Obesity (Silver Spring) 2018;26:1879-87. [Crossref] [PubMed]
- Rolls BJ. Dietary energy density: Applying behavioural science to weight management. Nutr Bull 2017;42:246-53. [Crossref] [PubMed]
- Kittrell H, Graber W, Mariani E, et al. Taste and odor preferences following Roux-en-Y surgery in humans. PLoS One 2018;13:e0199508. [Crossref] [PubMed]
- Abdeen GN, Miras AD, Alqahtani AR, et al. Vertical sleeve gastrectomy in adolescents reduces the appetitive reward value of a sweet and fatty reinforcer in a progressive ratio task. Surg Obes Relat Dis 2019;15:194-9. [Crossref] [PubMed]
- Nance K, Acevedo MB, Pepino MY. Changes in taste function and ingestive behavior following bariatric surgery. Appetite 2020;146:104423. [Crossref] [PubMed]
- Martin L, Jia C, Rouvelas I, et al. Risk factors for malnutrition after oesophageal and cardia cancer surgery. Br J Surg 2008;95:1362-8. [Crossref] [PubMed]
- Haverkort EB, Binnekade JM, Busch OR, et al. Presence and persistence of nutrition-related symptoms during the first year following esophagectomy with gastric tube reconstruction in clinically disease-free patients. World J Surg 2010;34:2844-52. [Crossref] [PubMed]
- Correia MI, Waitzberg DL. The impact of malnutrition on morbidity, mortality, length of hospital stay and costs evaluated through a multivariate model analysis. Clinical Nutrition 2003;22:235-9. [Crossref] [PubMed]
- Koizumi M, Hosoya Y, Dezaki K, et al. Postoperative weight loss does not resolve after esophagectomy despite normal serum ghrelin levels. Ann Thorac Surg 2011;91:1032-7. [Crossref] [PubMed]
- Boshier PR, Huddy JR, Zaninotto G, et al. Dumping syndrome after esophagectomy: a systematic review of the literature. Dis Esophagus 2017;30:1-9. [PubMed]
- Yamamoto K, Takiguchi S, Miyata H, et al. Randomized phase II study of clinical effects of ghrelin after esophagectomy with gastric tube reconstruction. Surgery 2010;148:31-8. [Crossref] [PubMed]
- Patel YC. Somatostatin and its receptor family. Front Neuroendocrinol 1999;20:157-98. [Crossref] [PubMed]
- Edmunds MC, Chen J, Soykan I, et al. Effect of octreotide on gastric and small bowel motility in patients with gastroparesis. Aliment Pharmacol Ther 1998;12:167-74. [Crossref] [PubMed]
- Fuessl HS, Carolan G, Williams G, et al. Effect of a long-acting somatostatin analog (SMS 201-995) on postprandial gastric emptying of 99mTc-tin colloid and mouth-to-caecum transit time in man. Digestion 1987;36:101-7. [Crossref] [PubMed]
- van Berge Henegouwen MI, van Gulik TM, Akkermans LM, et al. The effect of octreotide on gastric emptying at a dosage used to prevent complications after pancreatic surgery: a randomised, placebo controlled study in volunteers. Gut 1997;41:758-62. [Crossref] [PubMed]
- Foxx-Orenstein A, Camilleri M, Stephens D, et al. Effect of a somatostatin analog on gastric motor and sensory functions in healthy humans. Gut 2003;52:1555-61. [Crossref] [PubMed]
- Di Lorenzo C, Lucanto C, Flores A, et al. Effect of sequential erythromycin and octreotide on antroduodenal manometry. J Pediatr Gastroenterol Nutr 1999;29:293-6. [Crossref] [PubMed]
- Nelson-Piercy C. Effect of a new oral somatostatin analog (SDZCO611) on gastric emptying, mouth to cecum transit time, and pancreatic and gut hormone release in normal male subjects. J Clin Endocrinol Metab 1994;78:329-36. [PubMed]
- Kraenzlin ME, Wood SM, Neufeld M, et al. Effect of long acting somatostatin-analogue, SMS 201995, on gut hormone secretion in normal subjects. Experientia 1985;41:738-40. [Crossref] [PubMed]
- Parkinson C. A comparison of the effects of pegvisomant and octreotide on glucose, insulin, gastrin, cholecystokinin, and pancreatic polypeptide responses to oral glucose and a standard mixed meal. J Clin Endocrinol Metab 2002;87:1797-804. [Crossref] [PubMed]
- Kemmer TP. Inhibition of human exocrine pancreatic secretion by the long-acting somatostatin analog octreotide (SMS201-995). Aliment Pharmacol Ther 1992;6:41-50. [Crossref] [PubMed]
- Hopman WP, Wolberink RG, Lamers CB, et al. Treatment of the dumping syndrome with the somatostatin analogue SMS 201-995. Ann Surg 1988;207:155-9. [Crossref] [PubMed]
- Reasbeck PG, Van Rij A. The effect of somatostatin on dumping after gastric surgery: a preliminary report. Surgery 1986;99:462-8. [PubMed]
- Tulassay Z, Tulassay T, Gupta R, et al. Long acting somatostatin analog in dumping syndrome. Br J Surg 1989;76:1294-5. [Crossref] [PubMed]
- Geer RJ. Efficacy of octreotide acetate in treatment of severe postgastrectomy dumping syndrome. Ann Surg 1990;212:678-87. [Crossref] [PubMed]
- Gray JL, Debas HT, Mulvihill SJ. Control of dumping symptoms by somatostatin analog in patients after gastric surgery. Arch Surg 1991;126:1231-5. [Crossref] [PubMed]
- Hasler WL, Soudah HC, Owyang C. Mechanisms by which octreotide ameliorates symptoms in the dumping syndrome. J Pharmacol Exp Ther 1996;277:1359-65. [PubMed]
Cite this article as: Stratford NR, Murphy CF, Elliott JA, Reynolds JV, le Roux CW. Pharmacologic gut hormone modulation and eating behavior after esophagogastric cancer surgery: a narrative review. Ann Esophagus 2021;4:42.